This project aimed to design a thorium-based light water SMR to explore sustainable alternatives to traditional uranium fuel cycles. Motivated by thorium’s advantages in fuel availability, waste reduction, and proliferation resistance, the team used OpenMC and Scarabee to evaluate core configurations for reactivity, breeding performance, and thermal safety—advancing the potential of next-generation SMR designs
Team: Jonathan Trieloff, Trevor Howell, and Jaret Black
Project Advisor: Professor Hunter Belanger
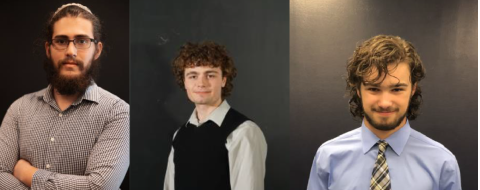
Members of the design group
Project Motivation
The motivation behind this project lies in addressing the limitations of traditional large-scale nuclear reactors—namely their reliance on scarce uranium resources, high capital costs, and limited portability. Small Modular Reactors (SMRs), particularly those based on the thorium fuel cycle, offer a promising alternative due to their potential for greater fuel availability, enhanced safety, and flexible deployment. This project aims to design a light water SMR (LWR-SMR) that leverages thorium's breeding potential, advancing sustainable fuel utilization through detailed neutronics and thermodynamic analyses.
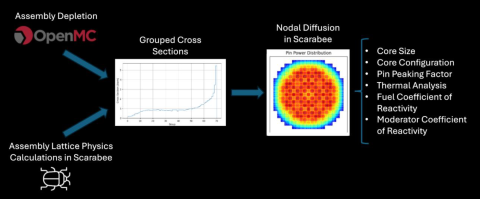
Workflow
Project Description
This project focuses on the conceptual and preliminary design of a light water small modular reactor (LWR-SMR) that utilizes the thorium fuel cycle. Aimed at achieving enhanced fuel sustainability and reducing reliance on conventional uranium, the reactor is designed to operate with a mixture of uranium dioxide (UO₂) and thorium dioxide (ThO₂), with uranium enrichment capped at 5%. The SMR targets a thermal power output of 600 MWth over 3 years and must maintain a negative temperature coefficient of reactivity for both fuel and moderator to ensure operational safety.
The project explores three distinct fuel configurations: (1) close-packed fuel pins with the maximum allowed uranium enrichment to enhance breeding potential, (2) standard-spaced fuel pins with a high thorium fraction to promote thermalization and breeding, and (3) layered fuel pins separating UO₂ and ThO₂ to examine geometric effects on performance. Using neutronic and thermal analysis tools such as OpenMC and Scarabee, each configuration is evaluated based on its criticality, breeding ratio, thermal performance, and proliferation risk.
The final design implements a MOX fuel configuration with up to 20% ThO₂ concentration, optimized pin pitch, and a full-core layout accommodating 311 assemblies to meet the 3-year fuel cycle requirement. Initially, the design slightly exceeded the limit for the moderator temperature coefficient of reactivity; however, a slight pin pitch reduction was successfully employed as a solution.
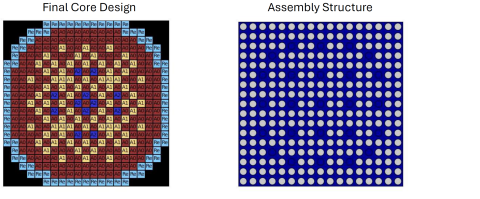
Final core design (left)and assembly structure (right)
Results and Accomplishments
The design project achieved the successful development of a thorium-fueled light water SMR that meets all specified technical requirements. Through extensive neutronic and thermal simulations, the final design demonstrated the ability to sustain a 3-year fuel cycle while maintaining a thermal power output of 600 MWth. The optimized fuel mixture—comprising up to 20% ThO₂ and 5% enriched UO₂—was shown to support substantial breeding of 233U from 232Th. Analysis confirmed that a significant fraction of the reactor’s power at end-of-cycle was derived from bred ²³³U, validating the feasibility of thorium as a sustainable fuel alternative.
Thermal performance met critical safety margins, with peak fuel centerline and cladding temperatures remaining well below their respective limits. Radial peaking was effectively controlled by strategically distributing assemblies with varying thorium content, resulting in a radial peaking factor of 1.56. The design also maintained a negative fuel and moderator temperature coefficient of reactivity, enhancing reactor stability. Although the moderator temperature coefficient was slightly positive at beginning-of-cycle, simulations showed that a minor reduction in pin pitch would resolve the issue, and this modification is used in the final design.